top of page
VICENTE AIGE GIL.DVM,PhD
Associate Professor of Anatomy · Facultad de Veterinaria
Universidad Autónoma de Barcelona
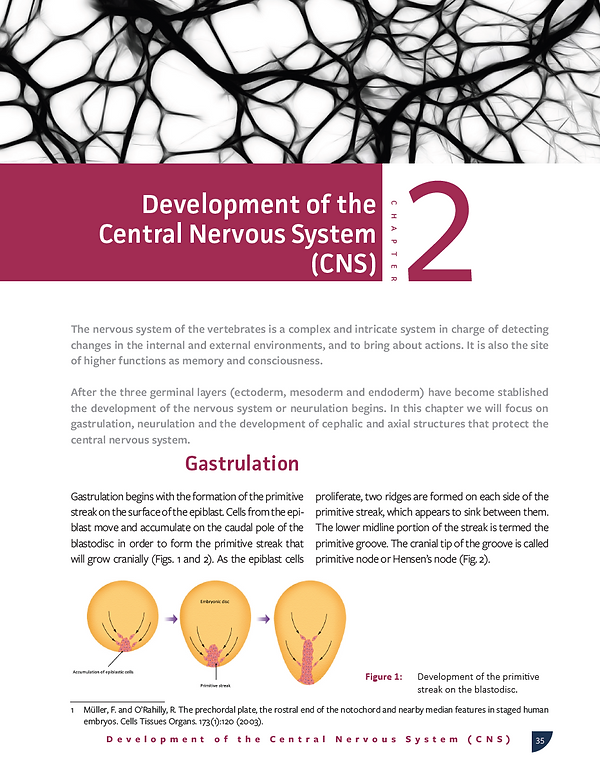



THE DEVELOPMENT OF THE NERVOUS SYSTEM
GASTRULATION
NEURAL TUBE FORMATION: NEURULATION
Primary neurulation
Neural tube defects
Secondary neurulation
ARCHITECTURE OF THE CNS TISSUE
Organization of the spinal cord
Organization of the encephalon
The cerebrum
The brain stem
The cerebellum
Choroid plexuses
ORGANIZATION OF THE PARAXIAL MESODERM
Vertebral malformations


bottom of page